The wearable for machine health: Condition-Based Monitoring
Published: 09 January, 2020
Condition-based monitoring (CbM) is the Industry 4.0 equivalent of wearable fitness devices. With the explosion of connectivity comes the opportunity to observe the physical world like never before and to see physical processes in action, in real time, in fine detail. In industrial systems, one of the processes important for us to understand is the process of the aging of equipment and machines. This is important in diverse markets from oil and gas, wind power generation, and industrial process control, where capital equipment costs are high, and downtime is costly. Stuart Servis*, senior applications engineer, reports.
Unplanned downtime can cost thousands of dollars per hour. One study in 2017 found that companies reported an average cost of $2 million due to downtime alone, and unplanned downtime is significantly more costly than planned maintenance because a machine must be offline while it’s diagnosed, parts are ordered, and repairs are made.
The continued operation of machines within specification, and the expected remaining lifetime of machines, is impacted by variables such as the time in operation, varying loads and operating environments, and damaging events. Condition-based monitoring seeks to quantify these impacts, provide alerts when immediate attention is required, and accurately predict when intervention will be required.
Every machine is different, and every machine will age in a different way, although the aging process is usually slow and imperceptible. Unless we actively look for indications of subtle changes over time, aging may go unnoticed for some time. Then suddenly there’s a failure, possibly catastrophic, that means that the machine is offline and in need of repair. End users are demanding earlier notice of impending failure to plan for downtime well in advance. They are also looking for indicators of more subtle changes in the machines that may affect the quality of their end product, such as paper and sheet metal.
The combined need for earlier and earlier indication of machine wear-out and information about machine output quality drives the need for more sensitive and more ubiquitous sensing. Measurement types are also broadening, with sensing modalities such as temperature and vibration being supplemented with acoustic, motor current, and voltage measurements. These measurement systems are being combined to give a more holistic view of the state of equipment. This leads to increasing numbers of measurement channels per machine. The individual measurements often need to be well synchronized to show relationships, such as between x-, y-, and z-axis measurements of vibration. This need for synchronization further increases the complexity of systems.
The increasing spread of measurement nodes and modalities means that manual inspection and measurement routines, based on manual resources, are no longer able to keep up. Systems must be deployable across the factory floor or remote site, with connectivity using existing wired infrastructure or wirelessly using robust and secure wireless systems. Bulky and expensive sensors and aggregator units must be smaller, cheaper, and more power efficient to fit in these environments.
There are new, precise solutions at the component and subsystem level with higher levels of integration to allow system builders to make this increased sensing future a reality now.
Data acquisition
To achieve the earliest possible indication of machine wear-out, you almost need to see into the future. In the realm of condition monitoring analytics, this is achieved by looking for the minutest changes in the system, whether it be system temperature, vibration, or acoustic signature. To sense these small changes requires sensors and data acquisition systems that can see the small changes clearly at the lowest detection levels, even amongst high levels of vibration or temperature. This requires signal chains with extremely high dynamic range, meaning systems with extremely low noise performance while still being able to deal with large variations in signal level. For example, to detect the onset of wear-out in a reciprocating pump may require the detection of a change in less than 1/10th of a mm of the end stroke position of the piston, where the piston is moving up to 300 mm overall. To ensure that we can see this change, the system noise must be below this by at least a factor of 10. This pushes the detection level to 1:300,000, or 109 dB, and requires 18-bit or more accurate data acquisition systems.
Another consideration is the need to push out of the bandwidth of interest. Motor axles and many gear systems have characteristic vibrations at relatively low frequencies, with frequencies at close to the rotation speed of the axle or low multiples of this. However, there are other components in the systems that have higher frequency features. To detect shifts in the wear of components that have higher frequency characteristics, such as ball and oil bearings, the sensing must be able to achieve high resolution and high dynamic range at frequencies beyond 10 kHz and up to 80 kHz.
The sensing system specifications must include high dynamic range (DR), as well as extremely low total harmonic distortion (THD) in order to resolve these frequency domain features in the system vibration profile. In these systems, the latest precision wide bandwidth sigma-delta (Σ-Δ) converters are used to perform the analog-to-digital conversion step. There are extremely accurate analog-to-digital converters that meet the key requirements for these systems. Converters in this category are specified with superior dynamic range and THD (typically +108 dB DR and −120 dB THD), which is achieved across a bandwidth of dc to at least 80 kHz, combined with ease-of-use features such as analog input pre-charge buffers, integrated digital filters, and cross-device synchronization for multichannel phase matching, make these key components in the building of the highest performance CbM data acquisition systems. Power scaling features allow the same physical hardware to be tuned to meet specific power ceilings, where dynamic range or bandwidth can be traded-off against total power. And providing accuracy at dc as well as wider bandwidth allows the input channels to address the needs of temperature, strain, and other dc or low bandwidth sensing in the same platform, which simplifies the overall condition monitoring system architecture and complexity—a single platform for all CbM sensor types.
Simultaneous Sampling
In CbM systems, simultaneous sampling is used to ensure the phase relationships between sets of time domain data is preserved. For example, where two orthogonally arranged vibration sensors are used, this allows the direction and amplitude of the vibration phasors to be detected. Ideally the phase delays through each sensor input path should be well matched and track over temperature.
For CbM systems that require even more flexibility in their design for wider range in their sampling rate, bandwidth, or power scaling needs, SAR ADC products are also appropriate. These devices also offer high dynamic range and THD, and at throughputs up to 2 MSPS, and also incorporate ease of use features that reduce signal chain power consumption, reduce signal chain complexity, and enable higher channel density. Converters with higher input impedance modes broaden the range of low power precision amplifiers that can drive these ADCs directly, while still achieving optimum performance.
To allow system builders to achieve the highest possible channel densities in more compact or distributed acquisition nodes, and to achieve faster time to market, signal chain μModule products with higher levels of integration than ever before are being developed.
These μModule devices combine key components commonly used in data acquisition signal chain designs within a compact, integrated circuit (IC)-like form factor.
The μModule approach transfers the design burden of analog and mixed-signal component selection, optimization, and layout from designer to device, which shortens the overall design time and system troubleshooting, as well as ultimately improves time to market. Housed in tiny packages, μModule devices are well suited to distributed low channel count, compact CbM systems or for higher channel count rack-based systems.
Sensors
Providing high dynamic range, wider bandwidths, greater power efficiency, and higher channel densities in the data acquisition part of the signal chain alone only addresses part of the system design challenge for CbM systems. Traditional integrated electronics piezoelectric (IEPE) vibration sensors are large, bulky, and expensive, and are usually run off relatively much higher voltage rails than the data acquisition system. Common piezoelectric sensors use a −24 V single supply, consuming upward of 2 mA, and are housed in heavy metal cases. Because the sensor supplies are usually provided by the data acquisition module, increasing the channel density in the box becomes a power density problem and a component density problem. Adding to this the need for wireless battery-powered acquisition nodes, the traditional piezo vibration sensor no longer meets the demands of these signal chains.
MEMS vibration and inertial sensors are now meeting the requirements of these systems. The latest wide bandwidth MEMS devices have noise and bandwidth performance that is ideal for CbM applications, and they achieve this performance in tiny standard surface-mount packages with power levels that are 20 times lower than comparable IEPE sensors. The small size and power profile of these MEMS sensors allow very small multiaxis, battery-powered systems to be developed for permanent and constant condition monitoring.
Power and connectivity
Sensing the temperature, vibration, or noise of the machine and converting this to digital information is a key part of the monitoring task, but these details do not provide the complete picture. To build condition monitoring systems requires paying close attention to all of the analog, digital, and mixed-signal components in the design. To achieve low noise in the data acquisition chain requires not only low noise sensors and analog-to-digital conversion components, but also low noise power design. And achieving low power in the system also requires power components that efficiently take power from the battery or field wiring without adding to the complexity of the design.
Connectivity needs will depend on the specific application environment. Many industrial facilities already have extensive wiring in place for process control or existing environmental sensing, such as temperature. However, much of this existing infrastructure may not be able to deal with the large amounts of raw data, or the data rates, required for extensive condition-based monitoring.
One approach is to enhance the capabilities of existing wiring by adding more data in ways that it does not affect the existing functions. For example, HART technology is used to add diagnostic information in digital form on top of the ubiquitous 4 mA to 20 mA analog interface. Similarly, Industrial Ethernet adds determinism and real-time control where existing Ethernet cabling exists, which allows for known latency in control applications as well as higher bandwidth where vibration or FFT data is required, as well as allowing multiple nodes on each link.
Another approach is to transfer the information wirelessly. In industrial environments, robust and secure wireless networking is required. The latest intelligent mesh radio products are wireless chips, and pre-certified PCB modules, which enable low power communication and >99.999% data reliability even in harsh, dynamically changing RF environments. For condition-based monitoring, this means that fault or transient events are guaranteed to be communicated to the host and can be acted on in the shortest possible time.
The Future of CbM
Condition-based monitoring is an absolute need for large capital cost equipment such as for energy and oil and gas, where unplanned downtime has a direct impact on production costs. It is also becoming more and more important on the factory floor where it can be used as both a proactive approach to machine maintenance, but also a way to ensure machines are producing product in a consistent way in normal operation. As the value of these monitoring capabilities becomes more apparent, this technology will start to be applied across more and more of the machines that we use every day—no longer the preserve of wind turbines or paper mills, we will see CbM in trains, planes, and automobiles, and eventually in washing machines and even smaller appliances.
Manufacturers of system components will integrate the sensor, or even the whole channel, into the component. Motors will come with vibration and current sensing included, and the same may become true for bearings and gearboxes. There will be self-contained sensor nodes that will report to your mobile device—deploy one on your garage door so it can warn you before your car gets stuck inside.
To meet the growing need for sensing in these many different scenarios, equipment makers will need to take a platform approach, where a smaller set of platforms can serve a more varied set of needs. Measurement channels will need to handle different sensor types so that rack-based equipment can be re-tasked for different combinations of sensors. In smaller equipment, the systems will need to be adaptable to different power profiles so that the same monitoring node can be used in a washing machine or a battery-powered tool.
Conclusion
Condition-based monitoring seeks to quantify the state of health of machines by sensing various measurable parameters within the machines. Increasing the accuracy and sensitivity of these measurements, as well as reducing the size, weight, and power of the monitoring equipment, allow the factory manager to deploy this sensing across the factory floor.
The factory now has a health monitor—a fitness tracker—that gives new levels of insight into the factory operations. Factory managers can be advised of minute changes in machine operation to make early and informed decisions with this information.
Scheduling maintenance in advance, and only planning it for those machines that really need it, can significantly reduce maintenance costs. After-hours call-out and on-call technician costs can be reduced to zero.
Capital equipment costs can be reduced due to the more controlled nature of the factory. Earlier detection and replacement of worn components protects the overall health of the machines. Closer monitoring reduces the occurrence of catastrophic failures. Equipment lifespans can be extended as the machines are carefully managed to end of life.
The cost of production of the factory end product can be reduced. With insight into machine health comes the possibility of maintaining control of the tolerances on the machines. End product output quality is more consistent from lot to lot. The occurrence of machine out-of-bound, or sudden stoppage, is reduced. Therefore, product rework and wastage are reduced.
For more information on condition-based monitoring solutions from Analog Devices, please visit www.analog.com/cbm.
*Stuart Servis is a product applications engineer at Analog Devices, where he works in the precision signal chains team, within the Instrumentation and Precision Technology Group. His area of expertise is precision data acquisition signal chains based on sigma-delta and SAR ADCs. He received his B.Sc. in applied physics and electronics from National University of Ireland, Galway.
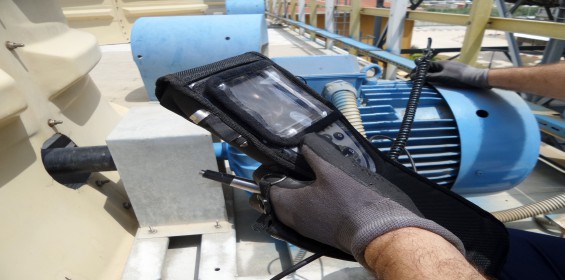